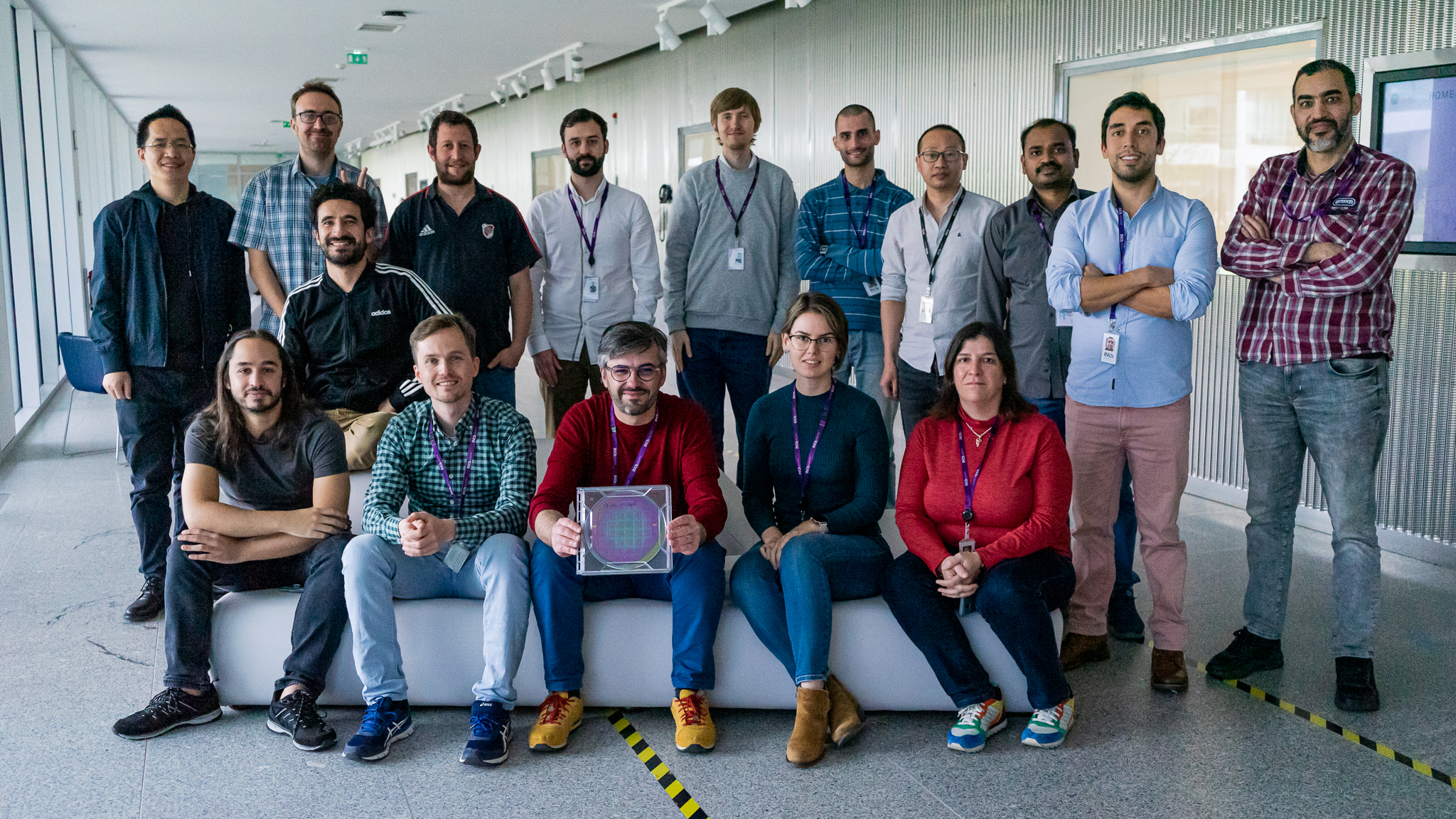
Spintronics
Spintronics is a research area which takes profit from the spin of the electrons as a means to obtain, transmit and process information. The spin of the electrons is a degree of freedom that is not explored by conventional electronics relying only on the electrical charge to drive electronic circuits. Spintronics use magnetic materials patterned at the nano-scale to produce spin-polarised currents which drive a new class of beyond-CMOS components which include magnetic field sensors, non-volatile memories and RF devices.
Research lines:
- Magnetoresistive sensors
- Spin dynamics and Spin Transfer Torque Nano-Oscillators.
Magnetic Annealing System (MATr 2000)
The MATR is a system used for annealing at elevated temperatures (up to 400 °C) in the presence of intense magnetic fields (up to 2 Tesla). Such magnetic fields are generated by a superconducting magnet module created by running current through windings of this material. The system can handle multiple wafers of 200mm and 150mm in diameter, in parallel or perpendicular position with respect to the magnetic field. There is also a 1×1 inch sample holder available.
UHV multi-target confocal sputtering tool (Kenosistec)
A multi-target UHV sputtering system consisting of a deposition chamber with 11 2” diameter magnetrons in confocal geometry for the co-deposition of materials, optimised for wafers of up to 200mm in diameter.
Ion Milling (Nordiko)
A broad ion beam milling system for 200mm wafers, designed to provide a very good within-wafer uniformity at slow etch rates to indiscriminately remove thin layers of material, with effective sample cooling. It is equipped with end-point detection using secondary ion mass spectroscopy (SIMS) for very precise milling.
TiW, AlSiCu, and Al2O3 Sputtering System (Timaris FTM)
The Four-Target-Module (FTM) physical vapour deposition cluster tool is specially designed for the deposition of high–quality metallic, conductive and insulating films. The system is a UHV single wafer cluster tool and consists of one transport module, one multi-target PVD module with up to four DC/RF cathodes (three targets are installed in this machine AlSiCu, TiW and Al2O3) and one soft etch/oxidation module. It is capable of depositing different magnetic and non–magnetic layers on wafers with diameters up to 200mm by DC/RF Magnetron Sputtering, with good uniformity for the deposited films. The FTM incorporates Linear Dynamic Deposition (LDD) technology in combination with up to four sputter targets in one vacuum chamber. The LDD technology enables the capability to deposit wedge films with a different film thickness across the wafer and to deposit alloy films with adjustable concentration gradients across one wafer.
Multi-target Sputtering Tool (Timaris MTM)
The Multi-Target Module (MTM) physical vapour deposition cluster tool is specially designed for the deposition of ultra–thin films, magnetic films, high–quality metallic, conductive and insulating films and multiple film stack deposition comprising these materials without the need to break ultra-high vacuum. The system is a UHV single wafer cluster tool and consists of one transport module, one multi-target PVD module with 10 DC/RF cathodes and one soft etch/oxidation module. It is capable of depositing different magnetic and non–magnetic layers on wafers with diameters up to 200mm by DC/RF Magnetron Sputtering (or Ion Beam Sputtering), with good uniformity for the deposited stacks.
Additional features such as wafer heating for hot substrate deposition or a collinear Aligning Magnetic Field (AMF) are available. The AMF can be activated to align the magnetic easy axis during the deposition of ferromagnetic films.
The Linear Dynamic Deposition (LDD) technology enables the capability to deposit wedge films with different film thicknesses across the wafer and to deposit alloy films with adjustable concentration gradients across one wafer. Both features allow a very cost-effective development of film stacks and accelerate device development.Current in-plane tester (CIPT)
To verify the transport properties of MTJs before nanofabrication a CIPT is used to perform TMR and RA measurements of bulk MTJ stacks. This is a very useful tool since it allows us to retrieve fundamental information of the MTJ stack prior to fabrication. To perform these measurements, the system contacts 12 cantilever electrodes with a variable spacing, down to 750 nm. It performs electrical measurements (current and voltage) through the different cantilevers with different spacing between them. This allows to determine the RA and TMR. The CIPT can determine RA values down to 0.1 Wμm² and measure the TMR with both in-plane and perpendicular anisotropy with in-plane fields up to 2500 Oe and perpendicular fields up to 1400 Oe.
Vibrating Sample Magnetometer (VSM)
The VSM allows the measurement of the magnetic moment as a function of the applied magnetic field of unpatterned samples. Thus, it allows us to measure and optimize the magnetic stack growth and magnetic annealings. The system can measure magnetic signals down to 5×10^-7 emu and very low coercivities (10 mOe; field resolution) and can apply fields up to 2 T. It allows fast and accurate measurements of the magnetic moment, not only as a function of the applied magnetic field but also with temperature (which can be swept from 83 to 570 K). Angular and time dependencies of the magnetization can be measured as well.
Automatic Transport Measurement Setup (CIPT)
Once the MTJ fabrication process is complete, this setup does a full characterisation of thousands of MTJ devices on the full wafer. A system with 40 tips, it can characterise 10 MTJs in a 4-contact scheme per landing site. Statistically meaningful data regarding the device TMR, RA, the shape of the transfer curves, and corresponding deviations arising from the nanofabrication process are obtained. Furthermore, the software allows the collection of different figures of merit (TMR, RA, coercive field, the linear range of the curve, etc) and organises them for different parameters (die number, pillar sizes, TMR and RA range, etc).
RF Prober for in-plane magnetic fields
Electrical contact to characterise RF devices was performed using special Cascade Microtech’s probes. These probes allow high-accuracy RF measurements with low contact resistance. It consists of an optical microscope in conjugation with holders that allow high-accuracy movements (both for the sample and the tips), used to connect the contacts to the device. The RF measurements can be performed while injecting a DC current in the MTJ.
Synchronization and spin diode torque studies can also be performed since an RF signal can be provided to the MTJ and the generated DC voltage measured. The signal is then transported through coaxial cables to a 3 Hz – 44 GHz spectrum analyser where the emission spectrum can be acquired. Power suppliers are used to provide current both to the MTJ and the magnet.
Automated control of the complete system can be performed to make sequences with different values of current and magnetic field. An amplifier is usually used to increase the measured signal, although its use was not necessary in the cases of MTJs with high output power. A bias tee is used to separate the DC and RF electrical components (being the last one sent to the spectrum analyser). The magnetic field was applied using a small magnet. The orientation of the magnet could be manually changed but it was limited to relatively small magnetic fields (up to 200 Oe) in the in-plane direction.
RF Prober for out-of-plane magnetic fields
Similar to the IP measurement setup, this is an RF measurement setup with power supplies and spectrum analyzer, but for out-of-plane magnetic fields. Magnetic fields up to 1.6 T can be applied. The magnetic field can be rotated between in-plane and out-of-plane directions with a highly precise stepper motor. The applied magnetic field value can be measured directly using a gaussmeter. The setup requires the positioning of the tips with the aid of an optical microscope. After the tips are properly connected to the contacts the microscope is easily displaced and the magnet is positioned in the sample position.
Projects
Publications
-
Non-Volatile Analog Control and Reconfiguration of a Vortex Nano-Oscillator Frequency
ARXIV, 2024The impact of local pinning sites in magnetic tunnel junctions with non-homogeneous free layers
COMMUNICATIONS MATERIALS, 2024Unbiased Random Number Generation using Injection-Locked Spin-Torque Nano-Oscillators
Physical Review Apllied (Accepted), 2024Non-hermiticity in spintronics: oscillation death in coupled spintronic nano-oscillators through emerging exceptional points
Nature Communications, 2024The Comprehensive Roadmap Toward Malaria Elimination Using Graphene and its Promising 2D Analogs
Advanced NanoBiomed Research, 2024 -
Classification of multi-frequency RF signals by extreme learning, using magnetic tunnel junctions as neurons and synapses
APL Machine Learning, 2023Weighted spin torque nano-oscillator system for neuromorphic computing
Communications Engineering, 2023Frequency Sensing and Detection using Granular Vortex MTJ Nano Oscillator
2023 IEEE International Magnetic Conference, 2023Method and system for non-invasive vibration-based condition monitoring of a machine
US Patent Office, 2023Spin-orbit torque flash analog-to-digital converter
SCIENTIFIC REPORTS, 2023Exploring Multifunctionality in MgO‐Based Magnetic Tunnel Junctions with Coexisting Magnetoresistance and Memristive Properties
Advanced Functional Materials, 2023A low-noise high-linear wide dynamic-range MTJ-based magnetic field sensor
2023 IEEE NORDIC CIRCUITS AND SYSTEMS CONFERENCE, NORCAS, 2023Granular vortex spin-torque nano oscillator for reservoir computing
SCIENTIFIC REPORTS, 2023Multilayer spintronic neural networks with radiofrequency connections
NATURE NANOTECHNOLOGY, 2023Second harmonic injection locking of coupled spin torque vortex oscillators with an individual phase access
Communications Physics, 2023Magnetic tunnel junction platforms for linear positioning and nanoscale displacement sensing
MEASUREMENT, 2023Unsupervised Extraction of Shape-based Signal Patterns for Incoming Signal Recognition
IEEE SENSORS JOURNAL, 2023 -
Design of Arbitrary Magnetic Patterns on Magnetic Polymer Composite Objects: A Finite Element Modelling Tool
POLYMERS, 2022Memory and Communication Logic (MCL) in Magnetic Tunnel Junctions
Trends in MAGnetism-PetaSpin Conference, 2022Spintronic Eyeblink Gesture Sensor With Wearable Interface System
IEEE TRANSACTIONS ON BIOMEDICAL CIRCUITS AND SYSTEMS, 2022Dual Band Radio Frequency Detector Based on the Simultaneous Excitation of Free and Reference Layer in a Magnetic Tunnel Junction
IEEE ELECTRON DEVICE LETTERS, 2022Effect of gallium doping on structural and transport properties of the topological insulator Bi2Se3 grown by molecular beam epitaxy
JOURNAL OF APPLIED PHYSICS, 2022Memory and Communication-in-Logic Using Vortex and Precessional Oscillations in a Magnetic Tunnel Junction
IEEE MAGNETICS LETTERS, 2022Spintronic Wireless Sensor Networks
IEEE TRANSACTIONS ON MAGNETICS, 2022Theoretical and experimental study of anomalous Nernst effect in biphasic magnetic system
JOURNAL OF MAGNETISM AND MAGNETIC MATERIALS, 2022Verilog-A-Based Analytical Modeling of Vortex Spin-Torque Nano Oscillator
IEEE TRANSACTIONS ON ELECTRON DEVICES, 2022A 3-Bit Flash Spin-Orbit Torque (SOT)-Analog-to-Digital Converter (ADC)
IEEE TRANSACTIONS ON ELECTRON DEVICES, 2022 -
Foldable layered connection, and method for manufacturing a foldable layered connection
US Patent Office, 2021Novel Polymorph of GaSe
ADVANCED FUNCTIONAL MATERIALS, 2021Developing a Data-Driven Unsupervised Pattern Recognition Approach for Sensor Signal Anomaly Detection
techrxiv, 2021Magnetoresistive Sensors and Piezoresistive Accelerometers for Vibration Measurements: A Comparative Study
JOURNAL OF SENSOR AND ACTUATOR NETWORKS, 2021Efficient ReSe2 Photodetectors with CVD Single-Crystal Graphene Contacts
NANOMATERIALS, 2021Hardware realization of the multiply and accumulate operation on radio-frequency signals with magnetic tunnel junctions
NEUROMORPHIC COMPUTING AND ENGINEERING, 2021Analog and Digital Phase Modulation and Signal Transmission with Spin-Torque Nano-Oscillators
PHYSICAL REVIEW APPLIED, 2021Beyond the gyrotropic motion: Dynamic C-state in vortex spin torque oscillators
APPLIED PHYSICS LETTERS, 2021Electrical characterisation of higher order spin wave modes in vortex-based magnetic tunnel junctions
COMMUNICATIONS PHYSICS, 2021Flicker and random telegraph noise between gyrotropic and dynamic C-state of a vortex based spin torque nano oscillator
AIP ADVANCES, 2021Phase variation in the locked state of mutually synchronized spin torque nano-oscillators
APPLIED PHYSICS LETTERS, 2021Radio Receivers based on Spin-Torque Diodes as Energy Detectors
2021 19TH IEEE INTERNATIONAL NEW CIRCUITS AND SYSTEMS CONFERENCE (NEWCAS), 2021Room temperature two terminal tunnel magnetoresistance in a lateral graphene transistor
NANOSCALE, 2021Van der Waals Epitaxy of Ultrathin beta-In2Se3 on Insulators Used in Standard Silicon Microelectronics Technology
CRYSTAL GROWTH & DESIGN, 2021Wafer-Scale Fabrication of 2D beta-In2Se3 Photodetectors
ADVANCED OPTICAL MATERIALS, 2021Non-volatile artificial synapse based on a vortex nano-oscillator.
Scientific Reports, 2021 -
Spintronic Sensors Based on Magnetic Tunnel Junctions for Wireless Eye Movement Gesture Control
IEEE TRANSACTIONS ON BIOMEDICAL CIRCUITS AND SYSTEMS, 2020Integrated Pico-Tesla Resolution Magnetoresistive Sensors for Miniaturised Magnetomyography
42ND ANNUAL INTERNATIONAL CONFERENCES OF THE IEEE ENGINEERING IN MEDICINE AND BIOLOGY SOCIETY: ENABLING INNOVATIVE TECHNOLOGIES FOR GLOBAL HEALTHCARE EMBC'20, 2020Magnetoresistive Sensor
US Patent Office, 2020Chemical instability at chalcogenide surfaces impacts chalcopyrite devices well beyond the surface
NATURE COMMUNICATIONS, 2020Detection of the Microwave Emission from a Spin-Torque Oscillator by a Spin Diode
PHYSICAL REVIEW APPLIED, 2020Digital and analogue modulation and demodulation scheme using vortex-based spin torque nano-oscillators
SCIENTIFIC REPORTS, 2020Eyelid Gesture Control using Wearable Tunnelling Magnetoresistance Sensors
2020 27TH IEEE INTERNATIONAL CONFERENCE ON ELECTRONICS, CIRCUITS AND SYSTEMS (ICECS), 2020LAO-NCS: Laser Assisted Spin Torque Nano Oscillator-Based Neuromorphic Computing System
FRONTIERS IN NEUROSCIENCE, 2020Ultrafast Sweep-Tuned Spectrum Analyzer with Temporal Resolution Based on a Spin-Torque Nano-Oscillator
NANO LETTERS, 2020Wideband High-Resolution Frequency-to-Resistance Converter Based on Nonhomogeneous Magnetic-State Transitions
PHYSICAL REVIEW APPLIED, 2020Thermopower measurements in magnetic nanowires
Woodhead Publishing Series in Electronic and Optical Materials, 2020 -
Cyber-Physical Production Systems supported by Intelligent Devices (SmartBoxes) for Industrial Processes Digitalization
2019 IEEE 5th International forum on Research and Technology for Society and Industry (RTSI), Florence, Italy, 2019Magnetodynamics in orthogonal nanocontact spin-torque nano-oscillators based on magnetic tunnel junctions
APPLIED PHYSICS LETTERS, 2019Magneto-mechanical actuation of magnetic responsive fibrous scaffolds boosts tenogenesis of human adipose stem cells
NANOSCALE, 2019Nanoscale true random bit generator based on magnetic state transitions in magnetic tunnel junctions
SCIENTIFIC REPORTS, 2019Portable sensing system based on electrochemical impedance spectroscopy for the simultaneous quantification of free and total microcystin-LR in freshwaters
BIOSENSORS & BIOELECTRONICS, 2019Spin torque nano-oscillator driven by combined spin injection from tunneling and spin Hall current
COMMUNICATIONS PHYSICS, 2019Spin-Torque-Nano-Oscillator based neuromorphic computing assisted by laser
2019 14TH IEEE INTERNATIONAL CONFERENCE ON DESIGN & TECHNOLOGY OF INTEGRATED SYSTEMS IN NANOSCALE ERA (DTIS 2019), 2019Spin-Orbit-Torque-based Devices, Circuits and Architectures
arXiv:, 2019 -
Influence of MgO Tunnel Barrier Thickness on the Output Power of Three-Terminal Spin Hall Nano-Oscillators
IEEE TRANSACTIONS ON MAGNETICS, 2018Influence of MgO Tunnel. Barrier thickness in 3-terminal Spin Hall Nano-Oscillators
2018 IEEE INTERNATIONAL MAGNETIC CONFERENCE (INTERMAG), 2018Broadband voltage rectifier induced by linear bias dependence in CoFeB/MgO magnetic tunnel junctions
APPLIED PHYSICS LETTERS, 2018Coherent control of acoustic-wave-induced magnetization dynamics in magnetic tunnel junctions
2018 CONFERENCE ON PRECISION ELECTROMAGNETIC MEASUREMENTS (CPEM 2018), 2018Excitation and coherent control of magnetization dynamics in magnetic tunnel junctions using acoustic pulses
APPLIED PHYSICS LETTERS, 2018Magnetic tunnel junction thermocouple for thermoelectric power harvesting
PHYSICS LETTERS A, 2018The magnetic tunnel junction as a temperature sensor for buried nanostructures
JOURNAL OF APPLIED PHYSICS, 2018 -
Thermal FMR Spectral Characterization of Very Low RA In-Plane MgO Magnetic Tunnel Junctions
IEEE TRANSACTIONS ON MAGNETICS, 2017Electrical measurement of absolute temperature and temperature transients in a buried nanostructure under ultrafast optical heating
APPLIED PHYSICS LETTERS, 2017Electrical measurement of absolute temperature and temperature transients in a buried nanostructure under ultrafast optical heating,
Applied Physics Letters vol.110, pp.232403, 2017Magnetic tunnel junctions with integrated thermometers for magnetothermopower measurements
JOURNAL OF PHYSICS-CONDENSED MATTER, 2017Spin transfer driven resonant expulsion of a magnetic vortex core for efficient rf detector
AIP ADVANCES, 2017Influence of the thermal interface resistance on the thermovoltage of a magnetic tunnel junction
PHYSICAL REVIEW B, 2017High power and low critical current density spin transfer torque nano-oscillators using MgO barriers with intermediate thickness
SCIENTIFIC REPORTS, 2017Towards High-Resolution Scanning Magnetoresistance Microscopy
2017 IEEE 12TH INTERNATIONAL CONFERENCE ON NANO/MICRO ENGINEERED AND MOLECULAR SYSTEMS (NEMS), 2017Flexible Magnetoresistive Sensors Designed for Conformal Integration
IEEE TRANSACTIONS ON MAGNETICS, 2017Influence of thermal gradients on the vortex dynamics in CoFeB MTJs
2017 IEEE International Magnetics Conference (INTERMAG), 2017